The potential molecular indicators of Parkinson’s symptoms
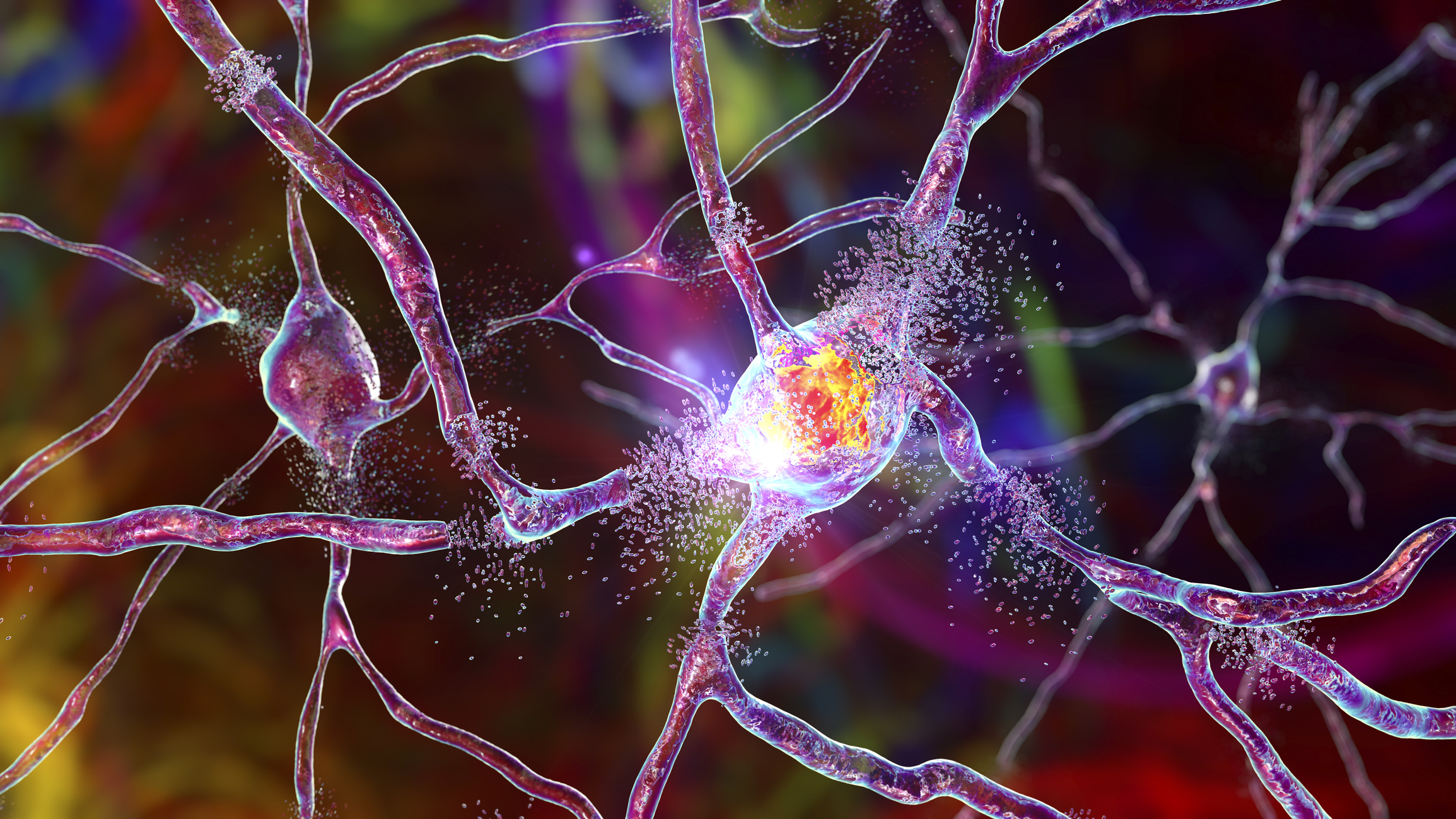
3D illustration of the destruction of neurons in the striatum, one of the brain regions affected by Parkinson’s disease
Hand tremors and slowed movements are two of the most widely recognized hallmarks of Parkinson’s disease, and for many sufferers the first indication that something is amiss. But by the time these symptoms manifest, those patients have already lost 50–80 percent of their dopamine neurons—a mass die-off that causes the neurodegenerative disease. Malfunctions in dopamine-dependent areas of the brain are responsible for many of the symptoms, which differ from person to person in an unpredictable way.
A diagnosis at an early age, typically before age 50, can stave off the most severe symptoms for years; when the disease is identified later, its trajectory is often swift and severe—but in lieu of symptoms, doctors have no tools for predicting the disease course.
Now scientists at Rockefeller University have made multiple discoveries that open new paths for the early prediction of disease trajectories and treatment of Parkinson’s. As published in Nature Communications, they found distinct RNA changes both in the blood of living Parkinson’s patients and in the brains of deceased Parkinson’s patients—and identified that there are many overlapping changes. These changes were further associated with many of the clinical symptoms of the disease.
The ability to detect molecular changes in the blood that mirror the changes in the brain could potentially be used to develop biomarkers that could predict a patient’s disease trajectory, allowing doctors to tailor treatment to the stage and symptoms of their disease, says first author Krithi Irmady, a movement disorders physician in the Laboratory of Molecular Neuro-oncology, headed by Robert B. Darnell. “We also think the molecular pathways we identified have great potential for pharmacological manipulation,” she says.
When dopamine neurons die
The second most common neurodegenerative disease after Alzheimer’s in the world, Parkinson’s is caused by the death of dopamine neurons in the substantia nigra, an area of the midbrain. Though often associated with feelings of pleasure, the neurotransmitter dopamine also helps to regulate motivation, memory, cognition, and movement.
One dopamine-dependent area connected to these essential functions is the striatum, located just above the substantia nigra. The striatum has two regions: the caudate and the putamen. Both are richly stocked with identical neurons, but they behave differently when it comes to Parkinson’s. When the caudate is starved of dopamine, cognitive impairment follows. When the putamen is, motor control goes off the rails. It’s a slow adaptive process, and the molecular reasons for these different responses have not been established in human brains.
Brain changes
In the first part of the study, the researchers sequenced RNA from the caudate and putamen of 35 people who’d died with Parkinson’s disease and compared those to 40 brains of neurologically normal brains as a control. They also delved into the clinical histories of those who’d had Parkinson’s to learn what their symptoms were and at what age they’d been diagnosed.
The Parkinson’s brains were brimming with RNA-driven changes in gene expression. And for the first time, researchers were able to link distinctly different molecular patterns in the striatum with patient symptoms.
“Patients with dementia had particular molecular patterns that were associated with the caudate,” Irmady says, including signaling mechanisms related to DNA packaging, which controls gene expression, and indicators of cellular stress.
In patients with involuntary jerky movements known as levodopa-induced dyskinesia—a known side-effect of levodopa, the main Parkinson’s medication—they found that the putamen’s endothelial cells, which form the lining of blood vessels, underwent molecular changes suggestive of self-destruction and vascular permeability, causing a kind of “leakiness.”
“It’s possible that when these blood vessels are affected differently in these patients, they have more access to the drug itself, causing too much medication in their brains,” Irmady says.
The caudate and putamen also showed distinct changes the longer someone had the disease. In the caudate, they found changes in the growth of the cells that form protective sheaths around brain nerves, while in the putamen they found distinctly different molecular changes suggestive of senescence, or accelerated aging of the cells. Because drugs that slow down cellular aging in Alzheimer’s patients are currently in clinical trials, “it was interesting for us to see that these pathways were affected in the putamen in Parkinson’s,” Irmady says. If the drugs prove effective for Alzheimer’s, perhaps a similar therapeutic approach could be taken for Parkinson’s.
There was also a marked difference between the brains of people who’d been diagnosed with Parkinson’s before age 55 and those who’d been diagnosed after. “The younger-onset patients had very modest changes of RNA compared to the controls, whereas the later-onset patients had thousands of genes that had changed,” Irmady says.
The findings, she says, “give molecular validation to what we sort of knew clinically: these patients are different. Now we know that their brains look different too.”
Blood ties
In the second part of the study, the team studied RNA from blood samples collected from 479 people in the Parkinson’s Progression Markers Initiative databank and compared them with 195 controls.
“We wanted to see what the overlap was between the brain and the blood,” Irmady says.
They found that hundreds of RNAs were significantly up- or downregulated in the blood of people with Parkinson’s—and remarkably, these RNA changes were altered in the same way in the caudate and the putamen. Many RNAs that would be expected to have a role in brain nerve-cell functions were in fact the most-altered RNAs detected in the blood. These nerve cell function–related RNAs also changed more in the blood with worsening disease severity. Similarly, RNAs associated with dementia in the caudate were also changed in the same direction in the blood of living patients with cognitive problems.
The researchers also found that the blood differed between those who had a younger onset versus a later onset of the disease. Just as the brains of later onset people with Parkinson’s disease showed more RNA changes, so too did the blood of living Parkinson’s patients.
Though they were “excited to notice this concordance between the blood and the brain,” Irmady says, “we don’t actually know why it happens. Are some of the RNAs from the brain just reflected in the blood, or is Parkinson’s a multisystem disease that has common patterns of gene expression across different systems of the body?”
Though such questions remain, Irmady has high hopes for the possibilities that could develop from their discoveries, including tools for predicting the course of the disease and new treatment avenues. “I think our findings will generate excitement about the promise of blood-focused studies for Parkinson’s disease,” she says.